
Highlighting Soil Conservation
Growing healthy soil is the foundation for growing healthy food. The purpose of this section is to promote practices that prevent soil erosion and build soil health. Several practices growers use to protect their soil also improve the quality of their crops and the well-being of the surrounding ecosystem. Many of the following practices are only necessary or suitable in certain situations such as in farming operations on a slope. Growers interested in implementing these operations should first contact the local NRCS or Resource Conservation District offices to understand the applicability of practices to their particular agricultural operation.

betterground.org
Soil Composition and Health
Soil is a living community comprised of inorganic and organic material as well as air and water (CCMG 2014). Soil organic matter (SOM) includes decomposing plant material, soil microbes, and humus, which is a product of organic matter decomposition (Veenstra et al 2006; Burgoa 2014). SOM improves the biological, chemical and physical properties of soil and provides readily available nutrients for plants and microbes. Properly managing SOM can increase nutrient availability to plants, and possibly reduce farmers’ reliance on fertilizers (Veenstra et al 2006). In healthy soil, SOM comprises about 5% of the soil. In addition, slightly acidic pH and a constant nutrient cycle throughout the soil system keep nutrients available to plants. Healthy soil also has strong granular structure with a mixture of pore sizes holding air and water (FAO 2005).
The life within soil creates its healthy structure. Soil organisms decompose organic matter, slowly producing humus which is highly resistant to further breakdown. Humus keeps nutrients available to plants (FAO 2005). Soil organisms also produce glues and filaments that bind tiny mineral particles and humus together into soil crumbs. Worms and other burrowing creatures continuously open pathways for roots, air and water. Good soil structure provides several benefits to farmers. It maintains critical soil air space while absorbing rain and irrigation water like a sponge. It drains excess water quickly, avoiding disease-friendly anaerobic conditions. It helps soil resist erosion and compaction and allows beneficial soil organisms to flourish. These organisms in turn maintain soil structure and facilitate the nutrient cycle. Soil tilth, a physical condition of soil indicating plant-growing suitability, relies heavily upon such structural qualities. Also affecting tilth are moisture content, degree of aeration, rate of water infiltration, and drainage (Boyle 1989/Sullivan 1999).
Soil Health Infographics. Source: NRCS Soil Health Awareness.
Nutrient Cycling
Nutrient cycle in the soil. Source: USDA-Agricultural Research Service, adapted from Brady, 1990, The Nature and Properties of Soils, 10th ed., p. 353, MacMillan Publishing Co., NY.
Regulating nutrient cycles within the soil is important for several reasons. Plants obtain nutrients from organic matter and minerals, so plant growth and yield depends highly upon the availability of these nutrients. The biological activity of different soil organisms depends on the organic matter content and composition. Most SOM comes from plant tissue and consists of carbon, oxygen, hydrogen, sulfur, nitrogen, phosphorus, potassium, calcium, magnesium and other plant-essential elements (FAO 2005; Burgoa 2014). SOM consists 10-40% of microorganisms and 40-60% of humus. The carbon to nitrogen ratio (C:N) indicates the ease of decomposition. For example, hard woody materials have a high C:N ratio and break down slower compared to soft leafy materials with a low C:N ratio. Nutrient exchanges between organic matter, water, and soil are essential to soil fertility. When farmers do not restore organic matter and nutrients, nor maintain good soil structure, nutrient cycles decline and soil fertility declines. To maintain the cycle, the rate of added organic matter from crop residues, manure, and other sources must equal the rate of decomposition and account for nutrient uptake by plants and nutrient losses from leaching and runoff. When organic matter accumulates in the soil faster than it decomposes—and faster than the rate of plant uptake, leaching and erosion—SOM increases (FAO 2005).
The following resources contain more information related to soil composition:
-
Building healthy soil, Contra Costa Master Gardeners, UCCE
-
Soil conservation as a method to prevent desertification, UNEP
Soil Erosion Principles and Trends
Erosion occurs when soil particles detach from raindrop impact or from surface runoff particularly if the runoff is concentrated (Casale 2014; Shanks et al 1998). It can also occur from wind, wave, glacial, gravity, volcanic, earthquake and other naturally occurring actions (Casale 2014). Soil erosion on farmland occurs when wind, irrigation water, or stormwater (when rainfall or irrigation application rate occurs at a rate faster than the soil’s water infiltration capacity) carries soil particles from a field in one of a few ways (Shanks et al 1998; Casale 2014). One is sheet erosion, which occurs when surface water flows over saturated soil and carries soil particles that were detached by the impact of raindrops. Rill erosion occurs when concentrations of surface runoff form small channels in the soil, called rills. Gully erosion occurs during intense rainfall on saturated soils or when rills merge into much larger channels (Shanks et al 1998). These types of erosion can also occur on non-saturated soil during intense rainfall events (Casale 2014). The erodibility of a soil from water action depends on factors such as the duration and intensity of rainfall, soil type, length and steepness of the slope, kind and condition of vegetative cover, and human impacts including the presence or absence of necessary management practices (Shanks et al 1998; Casale 2014; Burgoa 2014).
Sediment erosion on a relatively steep slope. Source: MCWRA, Reclamation Ditch Watershed Assessment and Management Strategy: Part A—Watershed Assessment, p. 88.
Erosion diminishes soil fertility, vital to sustaining plant growth, by removing topsoil, the layer of soil that is most rich in organic matter. In addition, water-borne erosion impairs water quality through the deposition and buildup of sediment in water bodies, causing pollution. Depending on several factors, such as the soil type, the number of cultivation during a period of time, timing, soil moisture and organic content, or the type of equipment, soil can become loosened or pulverized in a field. The most common factor is over-tilling the soil to the point that the soil structure is severely impacted and/or destroyed (Casale 2014). This makes soil particles more erodible, but it also removes vegetation that would hold soil in place and slow runoff to prevent the downhill movement of soil particles (Sonoma County Agricultural Commissioner’s Office). Without managing to protect soil and to slow, sink, or spread runoff, severe erosion can occur.
Even though farming can increase the risk of erosion and increase the rate and flow of runoff, such runoff rates are likely to be much lower than urban, industrial and transportation land uses that have higher proportions of impervious surfaces (Monterey County Agricultural Commissioner’s Office/RCDMC 2013).
Between 1982 and 2010, the state lost approximately 35% of its cropland to development, but California farmers have championed a wide array of practices to preserve the precious soil fertility on their remaining cropland. In 1982, state cropland lost almost 6 million tons of soil from water erosion at a rate of 0.83 tons per acre, plus over 7.5 million tons from wind erosion at a rate of 1.05 tons per acre, totaling an estimated 13.5 million tons of soil loss. Over the next few decades, California farmers streamlined irrigation efficiencies and improved soil management strategies, while also adopting practices to reduce the impact of blowing wind transporting soil from their land. Their efforts significantly reduced the rate of erosion (42%) and total soil loss (62%) statewide (derived from USDA 2013).
Erosion on cropland 1982-2010. Erosion from wind and water on U.S. cropland has reduced substantially since 1982. Source: USDA-NRCS, 2010 Natural Resources Inventory Summary Report, p. 7.
Policy Addressing Sediment Loading
Policies outlined to address sediment loading at the state level are part of the larger national effort to improve water quality, pertaining to the Clean Water Act (CWA). Section 303 (d) of the CWA requires states to list waters that do not meet water quality standards, and to develop total maximum daily loads (TMDLs) for those waters, accounting for all sources of the pollutants that caused the waters to be listed, including agricultural runoff. The Porter-Cologne Act (a California state law) requires each Regional Board of the State Water Resources Control Board to create and adopt water quality control plans for all areas within its region, and requires them to develop a program of implementation that describes methods to meet water quality standards, incorporating the TMDLs. TMDLs for sediment loading exist for the Pajaro River Watershed, part of which runs along the northern border of Monterey County.
-
TMDLs in Monterey Bay, wiki developed by ENVS 560/L Watershed Systems class at CSUMB
-
TMDL Program, State Water Resources Control Board
-
Sediment TMDL resolution for Pajaro River Watershed, Central Coast Regional Water Quality Control Board
Offsite sediment transport and deposition. Source: RCDMC, 2005, Water and Sediment Control Basin.
Conservation Tillage
Conservation tillage minimizes soil disturbance and preserves residues from the previous crop at the soil surface to reduce the risk of erosion. Counter to this is conventional tillage, which uses implements to mix and disturb the soil extensively, leading to a higher erosion risk. Conventional tillage leaves a rough soil surface, so growers must then disc the field smooth before planting (Plant and Soil Sciences eLibrary 2014). Generally in conservation tillage, growers reduce the depth and frequency of tillage operations, but can in addition vary tillage depths over time to avoid frequent soil disturbance at particular depths. Conservation tillage is especially useful in Monterey County on soils containing clay or a significant amount of moisture (RCDMC/MCAC 2013). In the Dust Bowl era during the formation of the Soil Conservation Service, the predecessor of the NRCS, agronomists determined that leaving 30% or more of the soil surface covered with plant residue was associated with significant reductions in the amount of soil loss from wind and water erosion, helping to end the crisis. Also effective is reducing the total passes through the field for tillage by 40% or more relative to customs in 2000 (Mitchell et al 2009; Mitchell et al 2012). Conservation tillage also saves growers time, as well as fuel and labor costs that accrue from disking and re-shaping beds.
Conservation tillage in Monterey County. A minimum till-ripper completes the final step of a minimum tillage program to increase fresh lettuce yields and reduce lettuce drop disease incidence. Source: Jackson et al 2002.
Growers in Monterey County experiment with varying conservation tillage methods to save topsoil and increase organic matter, to limit compaction and increase water retention, and to increase yields. Under no-till, growers leave soil undisturbed between harvest and planting except for possibly injecting fertilizers. The only disturbance occurs at planting, which is done by injection to make small holes in the soil via drills, coulters or seed disk openers. Shallow minimum tillage utilizes disks and lister bottoms to incorporate crop residue and cultivate the tops and sides of the beds in a single pass, then till at a shallow depth (~20 cm). Deep minimum tillage tills below the normal tillage depth at about 40 cm to push soil organic matter deeper into the soil to enhance growth of deeper-rooted plants and increase soil moisture. It includes operations known as deep plowing, sub-soiling, ripping, and row-till. In this operation, growers bury or mix soil deposits from wind or water erosion, reduce the concentration of soil contaminants that inhibit plant growth, and break up soil layers that prevent plant growth. Tillage choice depends on a number of variables such as soil type, crop type, and how this practice interacts with other on-farm practices.
Each tillage method has its own benefits and drawbacks. Over the past decades, local agricultural researchers conducted controlled experiments to determine the various agronomic impacts from using conservation tillage practices in combination with other agricultural conservation practices. In one study, researchers determined in lettuce and broccoli, two of the highest revenue crops in Monterey County, that combining no-till and deep tillage best sustained yields, and also reduced nitrate leaching and soil erosion. They highlighted that while no-till tended to reduce yields, it did not tend to increase pest problems. The researchers recommended that growers apply organic matter to the soil such as compost and a cover crop with each respective tillage method and alternate on a schedule between tillage methods such as deep tillage (Jackson et al 2004). In a similar study, researchers recommended that growers use shallow minimum tillage intermittently with deep minimum tillage to avoid disease and yield loss, based on the following observations: 1) continuous shallow minimum tillage increased total soil organic matter, and supported more microbial biomass and microbial diversity compared to deep minimum tillage; and 2) deep minimum tillage increased lettuce yields and decreased symptoms of lettuce drop disease compared to shallow minimum tillage (Jackson et al 2002). These recommendations echo the careful attention growers must pay to varying site-specific characteristics of their fields while considering options to manage for whole-farm health.
Supplemental conservation tillage implements. Top left: the Sundance System for shallow minimum tillage; bottom right: a minimum-till chisel for deep minimum tillage. Source: Jackson et al 2002.
The following resources explain advantages and disadvantages of using various tillage methods.
-
Deep tillage, NRCS
-
Minimum tillage practices affect disease and yield of lettuce, Jackson et al, 2002
-
On-farm assessment of organic matter and tillage management on vegetable yield, soil, weeds, pests, and economics in California, Jackson et al, 2004
-
No-tillage and high-residue practices reduce soil water evaporation, Mitchell et al, 2012
-
Tillage management, Plant and Soil Sciences eLibrary
Laser Land Leveling
In this land-smoothing process, tractors and soil movers are equipped with global positioning systems (GPS) and laser-guided instruments that allow soil to be moved either by cutting or filling to match soil to the desired contour and slope. A laser beam from a transmitter is intercepted by a receiver mounted on the leveling bucket. Then, a control panel mounted on the tractor interprets the signal, and opens or closes the hydraulic control valve which raises or lowers the bucket respectively. Loose soil is picked up by the bucket and released lower in the field. In most cases, fields need to be plowed and have topographic surveys conducted for them prior to leveling. After the process, fields usually do not need to be leveled again for several years (IRRI 2009).
This practice is particularly useful to increase irrigation uniformity in surface irrigation (e.g. furrow, flood, gated pipe), a group of irrigation types growers in Monterey County no longer practice, but it provides other benefits. To reduce water use, Monterey County growers refrained entirely from using furrow irrigation within the last five years (MCWRA 2012), but laser land leveling continues to provide benefits in other areas. Many of the fields in the Salinas Valley rotate over the years between different crops that require different tillage systems. To help reduce the loss of valuable and productive soil, many growers laser level fields in the lower-lying areas of the Salinas Valley to smooth them out. By smoothing and redistributing soil, growers can optimize the positioning of different topsoil types within the field that can be blown to other parts of the field or be eroded. Having a uniform soil surface also helps to achieve uniform crop establishment, growth, and maturity, leading to increased yields. In addition, the uniformity helps to alleviate standing water on fields. This can increase the uniformity of soil absorption and reduce weed pressure. Both the mechanization of smoothing a field and the benefits provided by the operation also enable farmers to reduce costs of labor which is in high demand due to the labor-intensive nature of managing specialty crops in the Salinas Valley.
Row Arrangement
Arranging rows along a more gentle slope helps water soak into the soil and increases irrigation uniformity. This slows runoff and leaves soil in place. When designing the layout of furrows, growers try to make the furrows as close to level as possible and try to prevent water pooling in the furrows that can damage both the furrows and the crops by avoiding slopes over 2%. In general, designs should avoid arranging furrows on slopes steeper than 4% or 5%. Growers design their layouts with existing soil types and soil conditions in mind. They map their fields and measure the slope of furrow lines to help decide how to lay out the furrows. The best option for steep areas and areas that carry a lot of water is to leave it in natural vegetation. In low spots that collect water growers incorporate the following into the design: a curve in the furrow to drain the low area; bisecting the block with a road through the low spot; PVC drain pipes to a furrow that carry water out to a road; and land leveling to fill the low area of the block and eliminate pooling. In cases where furrows become too steep, some designs include point or short rows to compensate.
Row Arrangements. Middle left: low point causes water pooling. Top and middle right: Curved rows reduce/eliminate pooling and reduce slope in some areas. Bottom left: Furrows are too steep. Bottom right: Point rows reduce slope. Source: RCD of Monterey County/Agricultural Commissioner’s Office, 2013, Hillslope Farming Runoff Management Practices Guide, p. 11.
The following resources serve as guides for growers to implement this practice.
-
Hillslope Farming Runoff Management Practices Guide, RCD of Monterey County/Monterey County Agricultural Commissioner’s Office, 2013
-
Furrow Allignment, RCDs and NRCS of Monterey and Santa Cruz Counties, 2003
Laser land leveling operation. The tractor hauls a scraper for leveling equipped with a laser receiver that guides the position of the cutting blade. The signal is received from the laser beam stand. Source: UCANR, 2013, “Land Formation”, In: California Rice Production Manual.
Most resources for this operation pertain to the benefits in a surface irrigated system. However, the following resources provide guidance on how to set-up and conduct this land preparation operation as well as make the case for high labor savings in furrow irrigation systems.
-
How to perform laser leveling, Rice Knowledge Bank, International Rice Research Institute (IRRI)
-
Manual for laser land leveling, National Agricultural Technology Project, Indian Council of Agricultural Research, 2002
Contour Farming
When farming on a slope, instead of planting rows up and down the slope, growers often farm along the curvature of the land in several contours to reduce sheet and rill erosion (Plant and Soil Sciences eLibrary 2014). This method has high potential to reduce erosion due to planting contours perpendicular to the slope, which reduces the length of the slope and thereby reduces the speed of runoff descending it. By slowing the flow of runoff, more of it infiltrates the soil. This method enables growers to best control runoff (USDA-NRCS YEAR) and is used in many of the hilly strawberry fields in northern Monterey County. A similar method is to align straight rows in myriad configurations across the slope to reduce runoff (see “Row Optimization” in Highlighting Water Conservation). This method is also a cost-saver, since reducing runoff reduces the size and costs for downstream runoff structures.
Contour farming in strawberry fields. Source: USDA-NRCS.
A drawback to contour farming is that it may be inconvenient or impractical for machinery or drip tape installation. In addition, the practice is not suitable for lands with heavy overland flows unless the flows can be diverted to safe outlets (USDA-NRCS).
The following resources provide more guidance on contour farming as well as discuss suitability and benefits and drawbacks of using the farming system.
-
Contour Farming practice description, USDA-NRCS
-
Contour Cropping, Plant and Soil Sciences eLibrary, 2014
Keyline Design
Keyline, key point, and keyline cuts on a slope. Source: Yeomans, P.A., 1954, The Keyline Plan, Sydney, Australia.
A lesser known practice is a whole farm-scale redesign known as keyline design. P.A. Yeomans developed it in Australia after noticing that rainwater runs across hillsides at the spot where hills transition from being ridges to valleys (Yeomans 1954; Hill 2006). The soil profile there results in it being a spot where water infiltrating into the soil seeps to the surface (Tunstall 2008). He determined that spot would be the highest point on the slope where a pond could most easily collect water to gravity-irrigate land below. He called this the keyline, and the point where it crosses the drainage lines, the keypoint (Yeomans 1954; Hill 2006).
Planting occurs in additional lines parallel to the keyline, instead of along contours, to divert surface flows from the normal drainage lines (gullies) toward ridges (Yeomans 1954; Tunstall 2008). The keylines distribute water more uniformly and increase its residence time on the field, thereby increasing the potential for infiltration, and maximizing water retention (Tunstall 2008). Placing retention basins at the keypoint enables growers to gravity-irrigate crops late in the season, bringing added benefits of delayed groundwater pumping and reduced pumping costs, as well as a reduced additional water requirement for their fields (Mamen and Dolman, 2008-2009).
Yeomans developed a special chisel plow for his system, known as the Yeoman’s plow. The ridge shoe of the plow lifts soil without inverting it, and combs lower soil layers. This aerates the soil profile and creates channels for water and nutrients, enabling deeper root growth and an effect that moves soil organic matter down deeper into the soil profile, ultimately improving the soil’s fertility and water-holding capacity (Tunstall 2008; Mamen and Dolman 2008-2009; Hill 2006).
This effect is related to the plowing technique and schedule, wherein plowing repeats for three years at increasing depths (e.g. 20 cm, 30cm, and 40cm or more depending on conditions in the deeper soil profile), ideally timed around warm weather and when expecting rain. This is contrary to conventional tillage that tends to decrease tillage depth over time due to compaction (Hill 2006). The method is designed to increase soil organic matter and microbial populations between plowing. Organic material falls down the plow lines and prevents their closure, increasing microbial populations deeper in the soil profile. These microbes then access new nutrients from soil minerals and the organic matter, and are sustained by the roots that exploit the fractures in the soil (Tunstall 2008). An attached vibration device helps break up compacted soil (hardpans), but also reduces resistance and enables the plow to be pulled by a lower horsepower tractor, reducing carbon emissions (Tunstall 2008; Hill 2006). The plow also does not bury vegetation (Tunstall 2008), and its narrow support shaft limits compaction (Hill 2006). Using this plow on his land, Yeomans was able to create 10 cm of topsoil in three years, whereas contemporary understanding was that such a process would take at least centuries (Hill 2006).
Yeoman’s plow and soil action. Source: R. Dofour, NCAT, 2010, Soil Building for Resilience, Powerpoint presentation.
Though the system was a major inspiration for the development of Permaculture (Mollison and Holmgren 1978), it remains under-utilized outside of Australia and under-studied in scientific literature. Few analyses of the system are avilable apart from the Yeoman family’s observations and academic literature mostly dealing with alternative agricultural systems. However, the system can be useful on compacted soils, given its potential to substantially increase infiltration and soil fertility, and to reduce erosion and runoff (Mamen and Dolman, 2008-2009; LandWatch Monterey County 2007). The system works best with other practices to increase organic matter, maintain cover on the soil, and promote healthy soil ecology. (Dufour 2010). Moving, storing and increasing infiltration of water on slopes using this design can potentially lead to slope instability and other erosion issues, so growers should consult with NRCS and/or a Certified Professional Erosion and Sediment Control Specialist (CPESC) before deciding on Keyline methods (Casale 2014).
The following resources inform growers on using this system.
-
Soil Building for Resilience, R. Dufour, National Center for Appropriate Technology (NCAT), 2010
-
Keyline Design, Mamen and Dolman, 2008-2009
-
The Keyline Plan, P.A. Yeomans, 1954
Terraces
Terraces are embankments constructed to provide a barrier for water flowing down a slope and are often built following the curvature of the land (Plant and Soil Sciences eLibrary 2014). Some Monterey County growers construct them along hilly areas in their vineyards and orchards to reduce erosion and sedimentation from runoff on steep slopes. If used in combination with select drainage practices, they can also greatly enhance water retention on farms. They reduce erosion by reducing the slope length and thereby reducing the velocity of runoff. They also increase water infiltration and reduce runoff that can erode soil (Plant and Soil Sciences eLibrary 2014). Excess water collected on terraces drains either through an underground pipe or through grassed waterways. Terraces also increases uniformity by planting on a level plane, which optimizes input use efficiency.
Terraced vineyard in Sonoma County. Photo: Lynn Betts, USDA NRCS, 2010.
Growers must use caution and should not implement this practice without consulting technical advisors. Building terraces can be expensive for farmers since it involves substantial soil moving and land grading to shorten the slope length. In addition, it’s not always suitable, and almost always has a destabilizing effect on slopes, especially when created by cutting and filling. Growers should especially avoid terracing in areas with inherent geological instability or where disturbances cut into steep slopes, as it could likely lead to slope instability. The steeper the slope is, the higher the likelihood of terrace and slope failure will be. Though terraces can help slow runoff and increase water infiltration, better infiltration on potentially unstable slopes can be disruptive and could lead to slope failure. This can also potentially lead to channeling and diverting of runoff, leading to gully erosion and other problems. Terracing requires additional practices and maintenance to operate as designed (Casale 2014). Growers should consult technical farm advisors at the local UC Cooperative Extension office or the Resource Conservation District and NRCS offices to ensure proper design of this conservation feature.
The following resources provide more information about the benefits of terraces and guidance on installing this feature.
-
Terracing, Plant and Soil Sciences eLibrary, 2014
-
Terrace conservation practice standard, USDA NRCS, 2010
Crop Rotation
Growing crops in a planned sequential rotation can add nutrients to the soil that may be diminished when growing one type of crop in a field over time. Crop rotation also has several other benefits. It can reduce erosion, improve soil quality, manage the balance of nutrients in the soil, fix nitrogen into the soil reducing energy use, conserve water, manage saline seepage and manage plant pests (NRCS 2011).
Crop rotation can reduce the incidence of Fusarium wilt, a disease in lettuce. UC Davis researchers observed that a crop rotation of resistant cultivars decreased the density of the inoculum that would infest a field by 86% within 1 year. By 34 months, the pathogen population reduced to 0.5% of that at the start of the field trial. Results demonstrated that damage from Fusarium wilt can be avoided by not growing a susceptible cultivar in a field with a history of the disease. The authors recommend growers reduce damage from the disease by using crop rotation and avoiding growing susceptible cultivars during the warmest planting windows, since disease susceptibility increased with soil inoculum density and temperature increases (Scott et al 2012).
The following resources describe more about crop rotation and offer further examples of crop rotation successes in the Salinas Valley.
-
Crop rotation description, NRCS, 2011
-
Broccoli residues can control Verticillium wilt of cauliflower, Koike and Subbarao, 2000
-
Crop rotation and genetic resistance reduce risk of damage from Fusarium wilt in lettuce, Scott et al, 2012
Crop rotation. Rotating plantings of different crop families incorporates multiple soil benefits over time. Source: ALBA Farmer Education Program Resource Guide, pp. 13-15.

Composting
Composting converts organic waste into a stable humus-like organic substance that makes nutrients more readily available to plants (EPA 2012; Burgoa 2014). It is an effective way to improve plant growth, because it enriches the soil with added nutrients. It enhances soil moisture retention, reducing runoff, erosion, and nutrient leaching, while aerating the soil (EPA 2012). It also improves soil tilth (workability) and sequesters carbon (CalRecycle). The presence of beneficial microorganisms in compost improves overall soil health and helps to control soil disease and pest infestation. Such beneficial uses increase healthy plant production, and reduce costs based on reduced use of chemical fertilizers (EPA 2012).
Recent research has encouraged growers to use compost based on its ability to increase yield. An economic analysis on a Salinas Valley farm observed that using cover crops plus compost in broccoli increased yields over those from non-amended soils to the extent that it provided a full return on investment within two years. Based on those observations, researchers highlighted that using cover crops plus compost is more economically viable compared to non-amended soils. These inputs also improved soil quality by increasing soil microbial biomass and decreased nitrate leaching (CIWMB 2002: 1).
Various forms of compost. Left to right: yard trimmings, steer manure, vermicompost, spent mushrooms. In trails: yard trimmings and mushrooms had higher microbial activity; manure best suppressed Verticillium wilt in strawberry; and vermicompost developed the deepest roots. Source: Lloyd 2014.
Researchers in Monterey County determined that composts made of municipal yard waste can increase yields in vegetable crops, especially in cases where cover crops are not possible or where scheduling only permits short-term cover crops (CIWMB 2002: 2). Composting facilities and waste collection agencies already collect yard waste to compost.
Another option is collecting residential food scraps to compost and distribute to growers to reduce the amount of food waste which accounts for 15.5% of total landfilled material in California (CIWMB 2009). Federal and state composting regulations require industrial composters to reduce pathogens and kill weed seeds in their products, but do not prohibit diverting municipal food scraps for use in compost production for agricultural fields. The extent of composting privileges depends on the permit issued to the composting facility (Sonoma Compost Company 2014). Currently, Industrial composting facilities must attain a full Compostable Materials Handling Facility Permit to compost food scraps (Title 14 CCR, Division 7, Chapter 3.1, Article 2, Sec. 17854), but concerns remain regarding contaminants from food scraps affecting the compost quality, as well as potential threats to food safety (CIWMB 2009).
These resources further inform residents and growers on how to safely and legally compost for soil health.
-
Compost effect on vegetable yield in Monterey County studies, CA Integrated Waste Management Board (CalRecycle), 2002
-
Composting for soil-borne disease control in Strawberry, Lloyd, 2014
-
Compost Use in Agriculture, CalRecycle
-
Composting Operations regulations, CalRecycle
-
Compostable Materials Handling Operations and Facilities regulations, CalRecycle
-
Monterey Regional Waste Management District compost sources and uses
-
The FSMA Produce Safety Rule: (see 2. Manure strategy to be further studied), US FDA, 2014
-
LGMA regarding the use of manures and composts, LGMA, 2014
Example of operations involved in a food scrap to field composting operation. Source: Jepson Prairie Organics/ Recology.
Cover Crops
Cover crops provide growers with a diverse chain of agronomic benefits. Planting cover crops is the most cost effective method to reduce the introduction of sediment, nutrients, and pesticides to stream channels through overland flow (SCAC 2014). Protecting bare soil surfaces is one of the best ways to prevent soil loss by water and wind erosion. The roots of cover crops encourage soil microbial biomass growth, which produces substances that increase soil aggregation to improve soil structure, improving water infiltration (SAREP). Certain rotations of cover crops can help control nematodes and many cover crops will attract beneficial insects. They also filter sediment, pathogens and pollutants attached to sediment. They can be temporary or long term, and perennial or annual plants (Central Coast RCDs 2012: 6).
Local research documents the success of using cover crops to control erosion and build healthy soil structure. In a study involving the use of cover crops in and between vine rows in Monterey County, plots of a type of triticale and rye cover crop increased soil organic matter and reduced sediment loss each year compared to bare ground plots. Significantly more microbial biomass
Cover crops in a vineyard. Left: Cover crops reduce runoff in a vineyard. Right: Absence of cover crops leaves vine rows susceptible to erosion during rains. Source: Smith et al., 2008.
accumulated under the rye than in bare ground, demonstrating its ability to boost soil productivity (Smith et al 2008). UC Cooperative Extension researchers in Ventura County are still investigating the runoff and sediment attenuation benefits of grass cover crops planted along hoophouse post rows, but their preliminary results indicate an observable benefit (RCDMC/MCAC 2013: 14).
Most important, growers should ensure that seed mixture provides overstory (tall fast growing plantsa like rye, grass, or barley) and understory (low growing broadleaf plants like clover) protection (SCAC 2014).
Cover crop management can also augment the nutrient supply in the soil. Growers often plant cover crops over the winter then mow and till them into the soil to add nutrients to the soil. The timing of nutrient release from the plant residue depends on various factors, such as its carbon to nitrogen ratio, and soil moisture, soil particle size, the method and timing of crop residue incorporation, soil nitrogen levels, and temperature. Legumes in a crop rotation form a symbiosis with nitrogen-fixing bacteria, fixing sufficient nitrogen for themselves and leaving a residual amount for the crops after them (Veenstra et al 2006; Burgoa 2014). The release of nutrients enables tilled-in cover crops to act as ‘green’ manure, enhancing soil fertility. This can potentially reduce the use of fertilizers. Cover crops, catch crops, and smother crops are all green manures. Catch crop examples include ryegrass and cereal cover crops (Veenstra et al 2006). Catch crops do not add nitrogen to the soil, but instead tie up nitrogen and prevent it from leaching into groundwater or surface water, reducing the degradation of groundwater quality
Barley and rye. Cover crops rotate with lettuce, broccoli, cauliflower, sweet peas, and seed beans. Source: Lance Cheung/USDA.
from building up high concentrations of nitrate. A smother crop is used to control weeds (NRCS 2005). One example of a smother crop is yellow mustard.
Improper cover crop management can cause problems for land managers, such as encouraging gophers, making trees more susceptible to frost, and increasing water use. However, when growers choose the right cover crop for their operation, they can minimize and even avoid these problems. For example, tall and dense stands of cover crops can lower nighttime temperatures and can lead to frost damage in vineyards and orchards. So, growers may want to consider either planting low growing cover crops or mowing their cover crops.
The following resources provide more information about the benefits as well as appropriate selection and management of cover crops.
-
Cover Crop Resources, Sustainable Agriculture Research and Education Program (SAREP)
-
Vineyard floor management affects soil, plant nutrition, and grape yield and quality, UCANR
-
In lettuce production, winter cover crops can decrease soil nitrate, leaching potential, Jackson et al, 1993
Soil Sampling
Soil sampling can help growers determine what nutrients to apply, how much, and when. Soil tests are essential to determine soil fertility and average nutrient status and availability in a field. Properly interpreting test results can assist growers in adjusting nutrient applications to increase yield, reduce production costs, and prevent surface and groundwater pollution (NRCS).
Sampling close to planting time helps most accurately estimate nitrogen availability, since some situations can cause soil values to change between sampling and planting, such as heavy rains that can leach nitrate-nitrogen from the root zone. Sampling at the same time each year is best for more consistent results. Nitrate-nitrogen concentrations should be determined annually for non-legume crops, and phosphorus and potassium determinations should be made every three to four years (NRCS).
Samples must be taken from appropriate depths to match lab test calibrations that distinguish between surface (0-6”) and subsurface (6-24”) samples. Surface soil samples are used to determine organic matter, phosphorus, potassium, pH, and salt levels. Subsurface samples are used to estimate available nitrogen and sometimes sulfur (NRCS). Newer soil testing methods can take into account the following soil biology traits to calculate soil health: soil N, P, and K; soil organic N and P; microbial activity; water extractable carbon (C); and C:N ratio (Haney 2013).
Collecting a representative soil sample in the field is critical to obtaining accurate test results. Non-representative areas, such as fences, roads, low areas, and salty or wet spots, should be sampled separately or avoided. Fields differing significantly in ways that affect nutrient availability, such as soil type, slope, drainage, and management history, should be divided and sampled separately.
Each group of samples should represent one uniform field area, wherein similar crop and fertility history, and similar soil characteristics exist. Sampling occurs in a random pattern, and at least 15-20 equal size cores to a minimum depth of 24” should be collected. Additional samples to a depth of 2-4’ may be needed in areas more vulnerable to leaching or where leaching highly varies (NRCS).
A stainless steel sampling probe is the tool of choice since it collects a continuous core, minimally disturbs the soil, and can be divided by sampling depth. To avoid contaminating samples, all tools should be clean, rust-free, stored away from fertilizers, and contain no galvanized material or brass (NRCS).
Each core is separated by surface and subsurface portions, which are placed in separate pails. Growers need to dry moist samples before bagging and sending them to a soil testing lab. It’s best to spread each sample on paper to air dry them at room temperature. They can also be bagged and frozen for shipping. Soil cores from at least 20 locations are thoroughly mixed in a clean plastic container, and approximately two cups of the soil mixture, known as the composite sample, are placed in a soil sample bag. Growers should send completed sampling information sheets (laboratories can often send upon request) with their samples to the lab that describe the location, past cropping and management history, proposed crops and tests requested (NRCS).
Biochar
The process of pyrolysis, essentially heating biomass in the complete or near absence of oxygen, produces biochar as one of its byproducts. Biochar differs from charcoal in that growers can use it as a soil amendment, however biochar contains little nutrient content. Instead, biochar improves soil fertility because its high surface area and porosity provide habitat to a host of microorganisms in the soil, making nutrients more available to crops (Schahczenski 2010). Biochar may also provide improved nitrogen and phosphorus availability to crops, reduce leaching, and enhance soil moisture retention, lessening the effects of drought, but these attributes depend on the method of production and feedstock used (Lehmann and Joseph 2009). Growers can apply biochar at a high application rate to balance the pH of soil when necessary, and thus provide a net carbon benefit by substituting out lime (Collins 2008).
Ongoing research strives for a standardized approach to the production of biochar to limit potential environmental problems associated with pyrolysis and biochar applications. Characteristics that determine the usefulness of the product or lessen the negative impact of production include whether producers used renewable feedstock, whether production increased greenhouse gas emissions, and whether biochar produced can improve soil quality in a reliable way (IBI 2009). In addition, the metal content and alkalinity can also enhance production impacts or affect product usefulness in improving soil fertility, but tests to determine the content of each are more difficult (Schahczenksi 2010).
Scientists determined that using the following feedstocks to produce biochar can help processors avoid diverting potential food sources and thereby avoid diminishing food security in areas depending on those crops: perennial plants grown on degraded lands abandoned from agricultural use; parts of crop residues from agricultural production provided that a significant portion is returned to the land to enhance future soil fertility and health; sustainably harvested wood and forest residues; double crops and mixed cropping systems that integrate food and dedicated fuel crops; and municipal and industrial wastes. Authors proposing such uses also underline the need to avoid large land use changes as a result (Tilman et al 2009).
Conservation burning and biochar production workshop (at right). Source: Sonoma Ecology Center, 2013, Mendocino County Biochar Workshop & Conservation Burn Training.
Local projects currently attempt to understand the potential agronomic benefits of biochar applications on farms. A Sonoma County nonprofit, The Sonoma Ecology Center, and the Sonoma County Water Agency implemented a project funded by a USDA NRCS Conservation Innovation Grant (CIG) to produce biochar from local wood wastes, field test its use and effectiveness at three local farms, and demonstrate its renewable energy capture and use (Sonoma Ecology Center 2013). The organization also partnered with the Community Alliance with Family Farmers, and the Farm Bureau to hold a conservation burn workshop conducting open burns that generate less pollution and produce biochar, featured at right. Another CIG project involves biochar application field trials implemented by the San Mateo County RCD on brussels sprouts to monitor crop yield and water quality and to analyze soil nutrients and soil organic matter over a three year period (POST 2012).
The following resources provide more information on the state of science regarding biochar research as well as updates on biochar projects.
-
Biochar and Sustainable Agriculture, J. Schahczenski, 2010
-
Sonoma County Biochar Project, Sonoma Ecology Center, 2013
-
Innovative Biochar Trials at Cabrillo Farms, 19 Jun 2012, Peninsula Open Space Trust (POST)
Sampling. Top to bottom: samples; sampling equipment; sampling depth. Sources: KSU Department of Agronomy; Central Coast Agricultural Water Quality Coalition; and KSU Soil Test Kit Information.
The following resources provide guidance on soil sampling.
-
Sampling Soils For Nutrient Management, USDA NRCS
-
Soil sampling, NRCS
-
Free soil sampling and resources on soil quality, McShane’s Nursery & Landscape Supply
-
Soil sampling for soil health, Haney, 2013, USDA-ARS
Yield differential example. Using biochar with nitrogen, phosphorus, and potassium fertilizer produced higher yields in this test than plain soil or fertilizer alone. Source: Carbon Zero Project, biochar.info
Pyrolysis process and product uses. Often, about 50% of the pyrolyzed biomass can return to the soil as biochar. Source: Lehmann, J., 2007, “Bioenergy in the black”, Frontiers in Ecology and the Envrionment 5: 381-7.
Buffer Strips and Vegetated Filter Strips
Conservation buffers are small areas or strips of land in permanent vegetation (trees, shrubs, grass) on farms. They can be especially useful near on-farm creeks, drainage ditches, reservoirs, and other perennial or intermittent streams (Central Coast Agricultural Water Quality Coalition, 2012; NRCS). Strategically placing buffers on a farm can effectively mitigate the movement of sediment, nutrients, and pesticides within and from fields. Buffers slow runoff, trap sediment, and enhance infiltration within the buffer (Massachusetts Department of Environmental Protection 2006).
They vary considerably in form and function. Field borders, vegetation planted in strips at the edge of fields, can be used for turn areas or travel lands for machinery. Riparian buffers are streamside planting of trees, shrubs, and grasses that can intercept pollutants from both surface and shallow groundwater before they reach a river or stream. They provide habitat for wildlife and enhance fish habitat by shading streams and creeks, thereby reducing water temperature. In areas with high salinity, growers can plant salt-tolerable vegetation that may ultimately help reduce saline seepage problems in the soil (CTIC 1999).
The main benefits of buffers include: soil and water quality improvement; fish and wildlife habitat enhancement and biodiversity protection; flood risk reduction; energy conservation; and protection of buildings, roads and livestock. Additionally, they can help trap fertilizers, pesticides, pathogens, and heavy metals, and reduce impacts from wind, dust, noise and odors. They can also effectively remove up to 50 percent or more of nutrients and pesticides, remove up to 60 percent or more of certain pathogens, and remove up to 75 percent or more of sediment. They often provide a source of food, nesting cover, and shelter for many wildlife species, and can provide corridors that enable wildlife to move safely from one habitat area to another. Buffers help stabilize streams and reduce water temperatures. They also offer a setback distance for agricultural chemical use from water sources (NRCS). If used as part of a comprehensive conservation system, buffers will make good use of areas that often should not be cropped (Massachusetts Department of Environmental Protection 2006).
Growers install vegetated filter strips down slope from crop rows to control erosion on the field, help remove sediment from runoff water in sheet flows, and to trap pollutants, such as phosphorus, and certain pesticides such as pyrethroids that normally become suspended in soil particles (RCDMC/MCAC 2013; Grismer et al 2006). In fact, these features can trap as much as 75 to 100 percent of sediment in runoff, and capture valuable nutrients that would otherwise leave the field. They also degrade and transform various pollutants to less-toxic forms and remove over 60 percent of certain pathogens from runoff (Grismer et al 2006).
Installing filter strips requires growers to consider several attributes, such as slope, filter strip width, and anticipated runoff. Filter strips are most effective on slopes under 10 percent at the lower field edge, areas not suitable for crop planting, and areas where runoff or sheet flow exist, especially next to bodies of water and drainages. These features can also be part of a riparian buffer (RCDMC/MCAC 2013). Growers should consult with technicians at the local NRCS and RCD offices to install these features and help determine strip width and effective locations for placement.
Buffer difference. Top: denuded buffers near Chualar along the Salinas River. Source: Sierra Club Ventana Chapter. Bottom: grass buffer strip on farm edge next to riparian forest provides soil and water quality protection. Source: Central Coast Agricultural Water Quality Coalition.
Filter strips. Top: separating crops from wetland area; bottom: buffering river from cropland. Source: RCDMC 2012.
Filter strips, like crops, require management and maintenance. Growers should provide necessary irrigation and soil amendments to ensure their effectiveness. Maintenance includes seasonal and frequent mowing to encourage dense growth and eventual removal and restoration once filter strips accumulate enough sediment (RCDMC/MCAC 2013).
The following resources provide more information on the benefits of buffers and filter strips, plus installation guidance.
-
Buffer Strips: Common Sense Conservation, USDA-NRCS
-
Conservation buffers fact sheet, Massachusetts Department of Environmental Protection, 2006
-
Vegetative filter strips for nonpoint source pollution control in agriculture, Grismer et al , 2006
-
Hillslope Farming Runoff Management Practices Guide, Monterey County Agricultural Commissioner’s Office/Resource Conservation District of Monterey County, 2013
Windbreaks and Windrows
Planting windbreaks to alter wind flow can help control soil loss from wind erosion and increase crop yield.
Windbreaks decrease wind speed and the width of the field exposed to strong winds, which reduces the rate of erosion and the damage to aggregates from saltating particles (particles carried by the wind for short distances) (Nordstrom and Hotta 2004). Moderately effective windbreaks can partially reduce normal wind speed for a distance of approximately 30 times the windbreak height (Borrelli et al 1989). This speed reduction is on average 60-80% near the windbreak downwind and out to a distance approximately 10 times the height of the windbreak, and about 20% at a distance approximately 20 times the barrier height (Tibke 1988).
Windrows. Top: Lines of trees protect grapevines from strong prevailing winds, common during the afternoon in the Salinas Valley. Source: Courtesy of Hahn family wines.
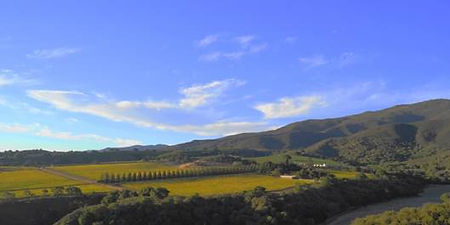
Generally, the shelter provided by windbreaks are correlated with average yield increases ranging from 6% to 44%, but research has not been able to isolate the effect that windbreaks have on yield due to other factors such as the effects of the microclimate on crop yield (Brandle et al 2004). Controlling wind erosion may also reduce the incidence and severity of crop diseases since wind-blown soil can carry inoculum for bacterial and fungal diseases and can provide pathways for pathogens to reach the field (Hodges and Brandle 1996).
Crop yield can significantly decrease in crops grown in close proximity to a windbreak, due to a number of effects, such as allelopathy (production of a chemical that reduces crop growth or reproduction), competition for nutrient or soil moisture, shading or temperature changes (Kort 1998). The magnitude of the effect varies by crop, windbreak species, location, and soil or climate conditions (Sudmeyer et al 2002). Under dry conditions, pruning the roots of the windbreak that extend into the field can reduce competition for soil moisture, and significantly increase crop yields. However, pruning must be repeated every one to five years and can diminish windbreak survival rates.
The following resources provide more information about windbreaks.
-
Windbreaks in North American agricultural systems, Brandle et al, 2004
-
Wind erosion from cropland in the USA: a review of problems, solutions and prospects, Nordstrom and Hotta, 2004
Grassed Waterways
Growers construct grassed waterways using the natural grade and strategically planted vegetation to convey runoff without causing erosion or flooding and to improve water quality. These also reduce wind erosion and help prevent the possible movement of pathogens via dust. They can stop sediment from entering waterways, reducing sedimentation and subsequent risk of flooding downstream (Central Coast RCDs 2012: 16-17). Grassed waterways are usually broad and more shallow. They enhance water infiltration and trap eroded sediment. They are less costly than losing soil to a ditch or stream, but they require periodic maintenance to work efficiently. They also help in preventing the development of gullies in the fields because the grasses are purposely established in the lowest part of the landscape, where concentrated water flow will likely occur (Plant and Soil Sciences eLibrary 2014).
The following resources provide more information on the benefits and implementation of grassed waterways.
-
Grassed waterways, Plant and Soil Sciences eLibrary, 2014
-
Conservation practice description, NRCS, 2010
-
Handbook of Conservation Agriculture Practices, Central Coast RCDs, 2012
Grassed waterway beneficial impact. Top: bare farm ditch prone to erosion; bottom: grass plantings slow runoff, stabilize banks, and filter out nutrients and sediment. Source: Central Coast Agricultural Water Quality Coalition.
Mulch, Wattles, Geotextile Fabric and Residue Management
On the Central Coast, ground coverings such as straw mulch, straw wattles, and geotextile fabric protect soil from erosion and complement various existing agricultural conservation features. Straw mulch can protect the soil during germination and enhance soil moisture retention and infiltration from rainfall or runoff in newly seeded areas of cover crops, vegetated filter strips, and grass roads (RCDMC/MCAC 2013; SCAC 2014; Casale 2014). Mulch also slows runoff, traps sediment, and over time increases soil organic matter (Casale 2014).
Mulching is a great emergency practice to implement in the winter when it’s too late to seed or in places where seed germination was unsuccessful. In these areas a thick layer (2 inches or more) of mulch by itself works great, but where mulch covers a seed bed, a lighter application (under 2 inches) is more suitable. Instead of covering several acres of cropland in mulch, growers can lay a buffer strip of mulch along the bottom of the slope and lay mulch strips approximately 10 feet wide perpendicular to the direction surface water flows over the slope, spaced every 50-100 feet depending on the length and steepness of the slope. Growers should lightly apply straw mulch that covers a seed bed to avoid burying the seeds too deeply, and they should break up the straw mulch flakes since seeds will not be able to grow through a dense layer of mulch.
Though rice straw tends to be “weed free”, it sticks together making it more difficult to apply, and depending on the amount of residual salt in or on the rice straw, seed germination can be significantly reduced. On some wind-prone sites, “tracking” or “punching” the mulch into the soil will help keep it in place (Casale 2014).
Straw or fiber mulch can also armor the ground where hoop-house anchors enter the soil, increasing the effectiveness of runoff and erosion control features added onto hoop-houses such as gutters and drains (RCDMC/MCAC 2013). Covering the soil surface along the sides of hoop-houses with geotextile fabric can also reduce erosion from rainfall impact and hold soil in place (RCDMC/MCAC 2013). Straw wattles are especially useful as barriers to reduce erosion from sheet runoff on short slopes and along stream banks. They slow runoff by shortening the slope length, and filter and trap sediment before reaching bodies of water. They must be installed along contours (SCAC 2014). These coverings also provide benefits in drainage systems. Geotextile fabric can provide additional stability in swales, and straw mulch can increase rainfall infiltration to recharge aquifers (SCAC 2014).
Straw mulch and wattles. Source: SCAC, 2014, Agricultural Erosion and Sediment Control BMPs, pp. 9, 11-12.
Straw wattle design. Source: SCAC, 2014, Agricultural Erosion and Sediment Control BMPs, pp. 9, 11-12.
Another option is to leave crop residues such as stems, leaves, and roots, on the ground following harvest to protect the soil from water and wind erosion. The degree of erosion control by residue cover depends on the residue type and amount (Plant and Soil Sciences eLibrary 2014).
The following resources provide cover rates and guidance on installing these features.
-
Residue Management, Plant and Soil Sciences eLibrary, 2014
-
Hillslope Farming Runoff Management Practices Guide, RCDMC/MCAC, 2013
-
Agricultural Erosion and Sediment Control BMPs, SCAC, 2014
Unlined grassed road. Source: RCDMC/MCAC 2013
Road Seeding
Since roads can cause severe erosion on farms, seeding grass on roads during winter can protect them and prevent erosion. This operation provides a large root mass that protects roads from washing out, protects bed ends from slumping, controls weeds, and improves water quality runoff into nearby streams. Growers should seed roads running directly downhill between planting blocks that capture and convey runoff downhill. Growers should make other determinations about the current flow of runoff on their land and take action to divert runoff away from roads even before seeding them to manage water before it reaches their roads. Growers should generally place roads in the natural low spots of the field and then arrange furrows according to the drainage capacity of the road. Growers may want to drain runoff from multiple blocks into a road that has existing drainage infrastructure and can provide more erosion control. On roads with less protection such as having only a grass and plastic-lined ditch, growers should orient furrows to drain runoff from one block onto one road, instead of concentrating runoff from multiple blocks onto one road.
More information on road seeding, including crucial considerations to make before seeding, is available in the following resource.
-
Hillslope Farming Runoff Management Practices Guide, RCDMC/MCAC 2013
Water Bars
Water bars help re-direct water from roads on steep slopes towards areas that can receive water safely to minimize erosion. They can also direct surface flows over roads to existing drainage structures or heavily vegetated areas. This practice is quick and inexpensive for growers to implement and can supplement road seeding when it is not feasible to install more permanent structures such as ditches and underground outlets.
Growers create water bars via cross-ripping, which creates fissures perpendicular to the slope for runoff to infiltrate and absorb into the subsoil. To cross-rip, growers excavate troughs at angles between 30 to 45 degrees downslope across the road. They pile excavated soil on the downhill side of the water bar to act as a levy, and to ensure that water does not bypass water bars, they connect the uphill end to ditches or furrows. It is best for growers to install protected outlets on the downhill end of the water bar to safely convey water to existing drainage structures or a naturally vegetated area on a more slight slope.
This practice is most suitable when roads are least travelled, such as in winter. When in use, growers should minimize traffic and establish vegetation on the water bars to further reduce erosion (RCDMC/MCAC 2013).
The following resource provides guidance on implementation and effective spacing for this practice.
-
Hillslope Farming Runoff Management Practices Guide, Resource Conservation District of Monterey County & Monterey County Agricultural Commissioner’s Office, 2013
Water and Sediment Control Basins
These basins collect excess sediment and runoff from fields. The slope of the receiving edge is designed to prevent erosion and maintain the basin structure. Within the basin, a vertical drainage pipe connects to a horizontal outlet pipe. An anti-flotation block anchors and connects the pipes beneath the basin. The basin slows and reduces the flow of runoff as it drains. The downstream side of the basin is comprised of a dike overlaying the outlet pipe (RCDMC/MCAC 2013; Burgoa 2014). Atop the dike, an emergency spillway provides a secondary drainage option if the basin overflows. Some growers install trash racks on risers atop the vertical pipes to collect debris and prevent it from entering drains. Designs should account for the anticipated rate and volume of runoff so as not to overwhelm the outlet while draining. To collect sediment only, growers typically install smaller basins (RCDMC/MCAC 2013).
Growers should consider installing basins at the low end of a field where runoff drains into ditches and underground outlets or where runoff can potentially overwhelm downstream structures. Growers should not construct these in stream channels or permanent water bodies. Basins should be large enough to hold the volume of sediment anticipated to be trapped at the site while the feature is in use, either for its useful life or for the length of time between clearing out the basin. It is best that a qualified engineer design the system after determining the anticipated peak flow runoff and desired outflow rates. To be effective, the design should accommodate sediment loads and excess runoff above natural predicted runoff. This will limit the potential for contaminated runoff to impair water quality, and will also reduce sedimentation, reducing flood risk (Central Coast RCDs 2012: 38-39). Growers should contact the Resource Conservation District of Monterey County for technical assistance in both the design and installation of this feature.
Water bar implementation. Top: Water bar cross-ripping example. Bottom: water bar spacing recommendations for unpaved roads. Source: RCDMC/MCAC 2013; Kocher et al 2007; Keller and Sherar 2003.
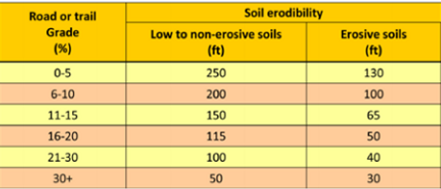
Water and Sediment Control Basin. Top: basin containing runoff and sediment; bottom: basic basin design. Source: Monterey County Agricultural Commissioner’s Office/RCDMC, 2013, Hillslope Farming runoff Management Practices Guide
The following resources provide more information on the design and management of water and sediment control basins.
-
Handbook of Agricultural Conservation Practices, Central Coast RCDs, 2012
-
Hillslope Farming Runoff Management Practices Guide, RCDMC/MCAC, 2013
Swale. Source: Permaculturevoices.com
Swales
A swale is a mildly sloping, shallow ditch that collects and stores runoff for a few hours or days between berms to let it infiltrate into the soil. Swales can be a cheap and easy solution to storing water and can be installed almost anywhere. In addition, trees and vegetation in swales can significantly increase water retention and further limit erosion from farmlands. This also allows pollutants to settle out of the water and cleanses water percolating down to the soil (SCMG).
The primary aim of installing swales is to trap and slow runoff flows, not to convey water. However, if the swale becomes full, the cleaner surface water will spill over the berm and slowly run into a nearby water body. Eventually, the remaining ponded water will either evaporate or infiltrate into the soil. Growers should consider installing swales in conjunction with other water retention and drainage practices, as well as incorporate rock riprap or vegetation designed to trap and slow runoff flows. They should prevent having compacted soil in the swale to encourage infiltration (SCMG; Burgoa 2014). Growers planning to add or change existing swales may need to obtain a Grading Permit from the Monterey County Planning Department. Some operations may be exempt if their plans allow for runoff below a certain flow standard measured in cubic feet per second (cfs) or if the excavated volume will be less than 100 cubic yards. In general, growers should design swales to handle runoff from at least a 25-year storm. Vegetated swales can be an alternative to drain pipe if they will more effectively slow and hold runoff. If installed on more than a minimal slope, swales may require reinforcement with geotextile fabric or grade control structures. Outlets from swales should incorporate features that minimize sediment delivery downstream, such as scour protection and energy dissipators (SCAC 2013).
The following resources provide guidance on swale installation and its benefits
-
Save the Swales, Sonoma County Master Gardeners, UCANR
-
Landscape Design to Minimize Runoff, Sonoma County Master Gardeners, UCANR
-
Best Management Practices for Agricultural Erosion and Sediment Control, Sonoma County Agricultural Commissioner’s Office (SCAC), 2013
-
The Grading Permit Process, Monterey County Resources Management Agency (MCRMA), Planning Department
Temporary slope drain. Source: Monterey County Agricultural Commissioner’s Office/RCDMC, 2013, Hillslope Farming runoff Management Practices Guide.
Temporary Slope Drains
This flexible pipe runs over the land and carries concentrated runoff from the top of a slope to its base without causing erosion. Water is diverted from a higher elevated area experiencing runoff and flows through the drain to a stable outlet and then into either a sediment or water control basin. Even though it is more difficult to install an underground outlet than a temporary slope drain, the opening at the drain’s uppermost point serves as its only inlet. These drains can be temporarily useful on lands that are not heavily used. They can also be used on lands that will not drain more than 10 acres and on slopes 3 percent or steeper that have not been stabilized. Multiple drains can be used in larger areas to divide the flow of runoff.
Several other considerations need to be identified before installing a temporary slope drain and growers wishing to do so should contact the local Resource Conservation District office.
The following resource provides guidance pertaining to the installation and maintenance for this practice.
-
Hillslope Farming Runoff Management Practices Guide, RCDMC/MCAC, 2013
Bank Stabilization
To remedy or prevent erosion along banks of streams or constructed channels leading to sedimentation, growers can try to stabilize banks along these areas. If needing to install bank stabilizing features, growers should contact the Resource Conservation District of Monterey County to utilize staff’s technical expertise and apply for USDA Farm Bill funding under an NRCS EQIP grant. Technical advisors customize the feature or combination of features to stabilize the area based on a number of site-specific considerations such as severity of slope, vegetation type, and the level of risk of erosion. Steps generally include: re-grading and re-vegetating the bank to eliminate overhangs and stabilize the slope; armoring the bank to control further erosion; and sometimes deflecting water flows away from vulnerable sites (Placer County RCD).
Bank stabilization protects banks of streams or constructed channels, and shorelines of lakes, reservoirs, or estuaries by stabilizing the ground to avoid soil erosion. It also prevents property damage to buildings or features near or downstream from bodies of water. It maintains the flow capacity of steams and channels and reduces the offsite or down-stream sedimentation resulting from bank erosion, improving and enhancing the stream corridor for fish and wildlife. Bank stabilization requires a permit due to complex issues such as local soil qualities, and environmental and hydrologic considerations (RCDMC 2012).
In stabilizing the bank, growers and technical advisors must ensure that nothing is done to heighten the potential for increased erosion, sediment loading in the stream, or runoff reaching and impairing the stream, given the sensitivity of the health of riparian corridors to such impacts. To reduce this risk, it’s advisable to use native plants that would be suitable for the surrounding area to stabilize the bank, and to avoid using invasive plants to prevent their spread. The Monterey Bay chapter of the California Native Plant Society may be able to assist in determining the native vegetation suitable for a given project.
The following resource provides management tips for streamside property owners to avoid erosion.
-
Stream Care Guide, Placer County RCD
-
Stream bank protection, Handbook of Agricultural Conservation Practices, RCDMC, 2012
Bank stabilization construction. Top: netting covers bare soil to reduce erosion and promote germination; bottom: netting with willow stakes above stabilizing features to protect erosion-prone site. Source: RCDMC 2012.
Bibliography
1.Bot, A., and Benites, J., (UN FAO), 2005, “The importance of soil organic matter”, FAO Soils Bulletin 80: Preface. Rome, Italy.
2.Borrelli, J., Gregory, J., Abtew, W., 1989, “Wind barriers—a reevaluation of height, spacing and porosity” Transactions of the American Society of Agricultural Engineers 32: 2023-27.
3.Boyle, M., Frankenberger Jr., W., and L. Stolzy, 1989, “The influence of organic matter on soil aggregation and water infiltration”, Journal of Production Agriculture 2: 209-99.
4.Brandle, J., Hodges, L., and X. Zhou, 2004, “Windbreaks in North American Agricultural Systems”, Agroforestry Systems 61: 65-78.
5.Burgoa, B., Resource Conservation District of Monterey County, personal communication, 5 Dec 2014.
6.CalRecycle, Organic materials management, Compost use in agriculture
7.Casale, R., Santa Cruz County NRCS, 2014, personal communication, 25 Nov 2014.
8.Central Coast Agricultural Water Quality Coalition, 2012.
9.Central Coast Resource Conservation Districts (RCDs), 2012, Handbook of Agricultural Conservation Practices, 3rd ed.
10.CIWMB, 2009, Food Waste Composting Regulations White Paper.
11.Collins, H., 2008, Use of biochar from the pyrolysis of waste organic material as a soil amendment: laboratory and greenhouse
analyses. From a quarterly progress report prepared for the Biochar Project.
12.Contra Costa Master Gardeners (CCMG), UCCE. Sustainable landscaping—building healthy soil.
13.CTIC, 1999. What’s a Conservation Buffer? Conservation Technology Information Center.
14.Grismer, M., O’Green, A., D. Lewis, 2006, Vegetative filter strips for nonpoint source pollution control in agriculture. UCANR publication 8195.
15.Haney, R., 2013, Soil Testing for Soil Health, PowerPoint for USDA-ARS, Temple, TX
16.Hill, S., 2006, “Redesign as deep industrial ecology: lessons from ecological agriculture and social ecology,” In: Cote, R., Tansey, J., and A. Dale (eds), Industrial Ecology: A Question of Design? Vancouver, BC: University of British Columbia.
17.International Biochar Initiative (IBI), 2009, Draft Guidelines for a Safe Biochar Industry. Proceedings of North American Biochar Conference. Boulder, CO.
18.International Rice Research Institute (IRRI), Cereal Knowledge Bank, 2009. Laser land leveling—a precursor technology for resource conservation.
19.ISU Extension, 1999, Management Systems Evaluation Areas, Water Quality, Jan 1999, “Strip intercropping,” Pm1763.
20.Jackson, L., Ramirez, I., Morales, I., and Koike, S., 2002, “Minimum tillage practices affect disease and yield of lettuce,” California Agriculture 56(1):35-40. DOI: 10.3733/ca.v056n01p35.
21.Jackson, L., Ramirez, I., Yokota, R., Fennimore, S., Koike, S., Henderson, D., Chaney, W., Calderón, F., and Klonsky, K., 2004, “On-farm assessment of organic matter and tillage management on vegetable yield, soil, weeds, pests, and economics in California,” Agriculture, Ecosystems and Environment 103:443-63.
22.Kort, J., 1988, “Benefits of windbreaks to field and forage crops”, Agriculture, Ecosystems and Environment 22/23: 165-90.
23.Lehmann, J. and S. Joseph, eds., 2009, Biochar: Environmental Management. Earthscan, U.K. and U.S.
24.Mamen, K., and B. Dolman, California Agricultural Water Stewardship Initiative (CAWSI), 2008-2009, Keyline Design.
25.Massachusetts Department of Environmental Protection, 2006, Conservation buffers fact sheet, In: Chapter 10: Agriculture, Massachusetts Nonpoint Source Pollution Management Manual, The Clean Water Toolkit, prepared by GeoSyntec Consultants.
26.Mitchell, J., Pettygone, G., Upadhyaya, S., et al 2009, “Classification of conservation tillage practices in California irrigated row crop systems”, UCANR Publ. 8364, Oakland, CA, 8 pp.
27.Mollison, B. and D. Holmgren 1978. Permaculture One: A Perennial Agriculture for Human Settlements. Melbourne.
28.Nordstrom, K., and S. Hotta, 2004, “Wind erosion from cropland in the USA: a review of problems, solutions and prospects”, Geoderma 121: 157-67.
29.Placer County Resource Conservation District (RCD), 2014. Stream care guide: management tips for streamside property owners—addressing stream bank problems.
30.Plant and Soil Sciences eLibrary (PASSEL), USDA, 2014.
31.Schahczenski, J., National Center for Appropriate Technology (NCAT), National Sustainable Agriculture Information Service (ATTRA), 2010, Biochar and Sustainable Agriuclture, 12 pp.
32.Scott, J., Gordon, T., Kirkpatrick, S., Koike, S., Matheron, M., Ochoa, O., Truco, M., and R. Michelmore, 2012, “Crop rotation and genetic resistance reduce risk of damage from Fusarium wilt in lettuce”, California Agriculture 66(1): 20-24.
33.Shanks L., Moore D., and C. Sanders, 1998, “ Soil erosion”, In: Cover Cropping in Vineyards. A Grower’s Handbook. 80-85. Ingels C.A., Bugg R.L., McGourty G.T., Christensen L.P. (eds.), University of California. Publication 3338. Oakland. USA.
34.Smith, R., Bettiga, L., Cahn, M., Baumgartner, K., Jackson, L:., and T. Bensen, 2008, “Vineyard floor management affects soil, plant nutrition, and grape yield and quality”, California Agriculture 62(4): 184-90. DOI: 10.3733/ca.v062n04p184.
35.Sonoma Compost Company, 2014, personal communication with Pam Davis, 12 Sep. 2014.
36.Sudmeyer, R., Hall, D., Eastham, J., and M. Adams, “The tree-crop interface: the effects of root pruning in south-western Australia”, Australian Journal of Experimental Agriculture 42: 763-72.
37.Sullivan, P., 1999, “Soil tilth and organic matter”, In: Sustainable Soil Management Soil Systems Guide, Appropriate Technology Transfer for Rural Areas (ATTRA).
38.Tibke, G., 1988, “Basic principles of wind erosion control”, Agriculture, Ecosystems and Environment 22/23: 103-22.
39.Tilman, D., et al 2009, “Beneficial biofuels: the food, energy, and environmental trilemma”, Science 325: 270-1.
40.Tunstall, B., 2008. “Degradation of Farming Systems”, Environmental Research and Information Consortium (ERIC), 14 pp.
41.US EPA, 2012, “Composting,” epa.gov Ag Center
42.USDA NRCS New York, “Deep tillage,” Practice code 324.
43.USDA NRCS, 2005, “Use of manures or legumes for nutrients,” Conservation activity job sheet.
44.USDA NRCS, 2011, “Conservation crop rotation,” Conservation practice standard code 328.
45.USDA NRCS, “Buffer strips: common sense conservation,” webpage on NRCS website.
46.USDA NRCS, “Sampling Soils for Nutrient Management”, pamphlet available on NRCS website.
47.USDA NRCS/United States Forest Service (USFS), National Agroforestry Center, 2014, “Windbreaks”.
48.USDA NRCS, “Soil testing,” Reproduced by NRCS Indiana and Indiana Grazing Lands Conservation Initiative.
49.USDA. 2013. Summary Report: 2010 National Resources Inventory, Natural Resources Conservation Service, Washington, DC, and Center for Survey Statistics and Methodology, Iowa State University, Ames, Iowa.
50.Yeomans, P., 1954, The Keyline Plan, Waite & Bull, Sydney.
51.Veenstra, J., Horwath, W., Mitchell, J., Munk, D., 2006. “Conservation tillage and cover cropping influence soil properties in San Joaquin Valley cotton-tomato crop”, California Agriculture 60(3):146-53. DOI: 10.3733/ca.v060n03p146.
Created with Wix.com by Brent Hassebrock in 2015